Energy Efficiency in HVAC Systems
Energy efficiency is a cornerstone of modern HVAC system design, driven by rising energy costs, environmental responsibility, and increasingly stringent regulatory requirements. Heating, ventilation, and air conditioning typically account for 40–60% of a commercial building’s total energy consumption. As such, optimising HVAC performance has a significant impact on both operational expenditure and environmental footprint. Compliance with energy codes such as NCC Section J, Green Star, NABERS Energy, and AS/NZS 3598 requires an integrated design approach that considers system selection, control strategies, zoning, thermal loads, and building envelope performance. Beyond compliance, energy efficiency is central to achieving net-zero carbon targets and long-term sustainability.
System Selection and Part-Load Performance
Energy-efficient HVAC begins with selecting systems suited to the building’s thermal load profile, climate zone, and usage pattern. Variable Refrigerant Flow (VRF) systems, water-cooled chilled water systems, and hybrid air conditioning systems are often chosen for their part-load efficiency and controllability. Integrated Part Load Value (IPLV) and Coefficient of Performance (COP) are used to assess performance at off-design conditions, which represent the bulk of a system’s operating life. For example:
- A high-efficiency air-cooled chiller might have a COP > 3.2 and an IPLV > 4.5.
- Water-cooled chillers may achieve a COP > 6.0 under optimal conditions with cooling tower heat rejection.
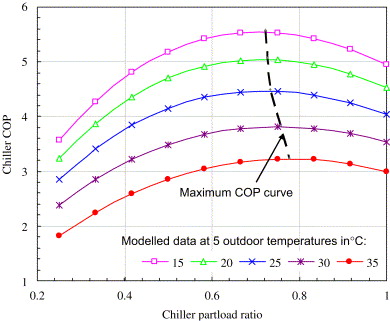
Proper selection must consider not only nominal efficiency but also performance under low-load, variable ambient, and turndown conditions. Designers often model seasonal energy performance using simulation software (e.g. IES VE, eQUEST, EnergyPlus) to evaluate different configurations across an entire year.
Air-Side and Water-Side Energy Recovery
Energy recovery systems reclaim waste energy to pre-condition supply air or chilled/hot water, significantly reducing primary HVAC energy demand. On the air side, enthalpy wheels, plate heat exchangers, or run-around coils transfer heat and moisture between exhaust and supply streams. These systems can recover 50–80% of sensible energy and 30–60% of latent energy, depending on the device type and flow conditions.
Water-side energy recovery includes:
- Condenser water heat recovery from water-cooled chillers to provide domestic hot water.
- Heat reclaim chillers that simultaneously provide chilled and hot water with COPs > 6.
- Run-around loop systems transferring energy between remote air-handling units.
Recovery devices must be modelled for pressure drop, fouling factor, bypass control, and maintenance access to ensure long-term efficiency and compliance with AS/NZS 3666 for hygiene.
Zoning and Load Management
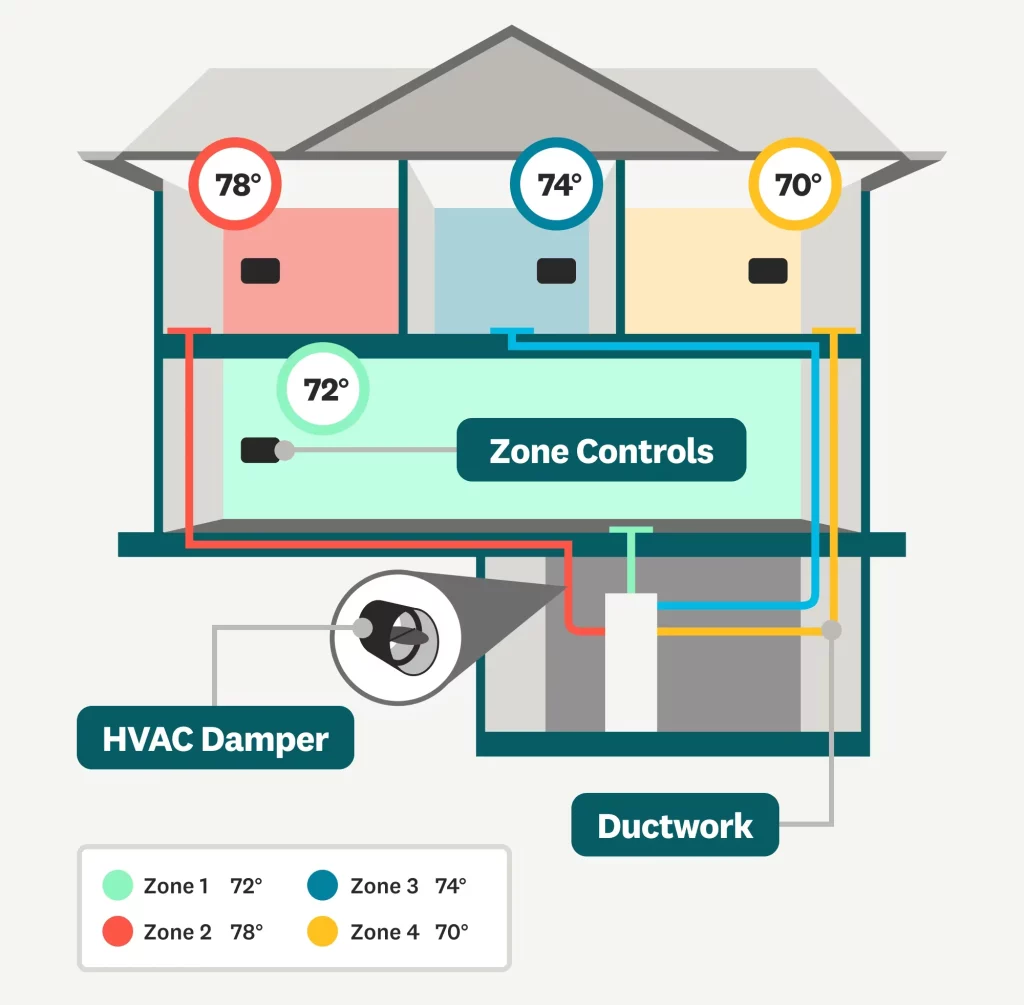
Zoning HVAC systems improves controllability and reduces simultaneous heating and cooling. This is particularly important in mixed-use buildings with diverse internal loads and varying occupancy patterns. Zoning strategies may include:
- Terminal unit control with VAV boxes or FCUs.
- Dedicated outdoor air systems (DOAS) to decouple ventilation from thermal conditioning.
- Thermal zoning by orientation to address solar gains.
Load diversity should be accounted for in central plant sizing, avoiding oversizing which leads to inefficient part-load operation. Thermal diversity schedules are applied during simulation to model realistic load profiles across zones.
Controls, BMS Integration, and Optimisation
Building Management Systems (BMS) play a critical role in energy optimisation by enabling real-time control, sequencing, and scheduling. Effective control strategies include:
- Setpoint resets for chilled/hot water based on outside air temperature or zone demand.
- Economy cycle control (free cooling) when ambient conditions permit.
- Demand-based ventilation to vary outdoor air flow based on occupancy.`
- Static pressure reset in VAV systems using fan speed control.
Advanced analytics platforms can perform fault detection and diagnostics (FDD), identify inefficient operation (e.g., simultaneous heating and cooling), and optimise control loops. BMS systems must comply with interoperability standards (e.g., BACnet or Modbus) and integrate with energy metering systems to report on NABERS energy usage intensity (EUI) and performance.
Thermal Insulation and Envelope Considerations
Envelope performance significantly affects HVAC efficiency by influencing peak loads and energy consumption. Section J of the NCC specifies minimum thermal insulation (R-values) for walls, roofs, and glazing, with performance values based on building classification and climate zone. Common strategies include:
- High-performance glazing with low solar heat gain coefficient (SHGC).
- Thermal breaks in curtain walls to reduce conductive losses.
- Roof insulation systems with vapour barriers to prevent condensation and latent load infiltration.
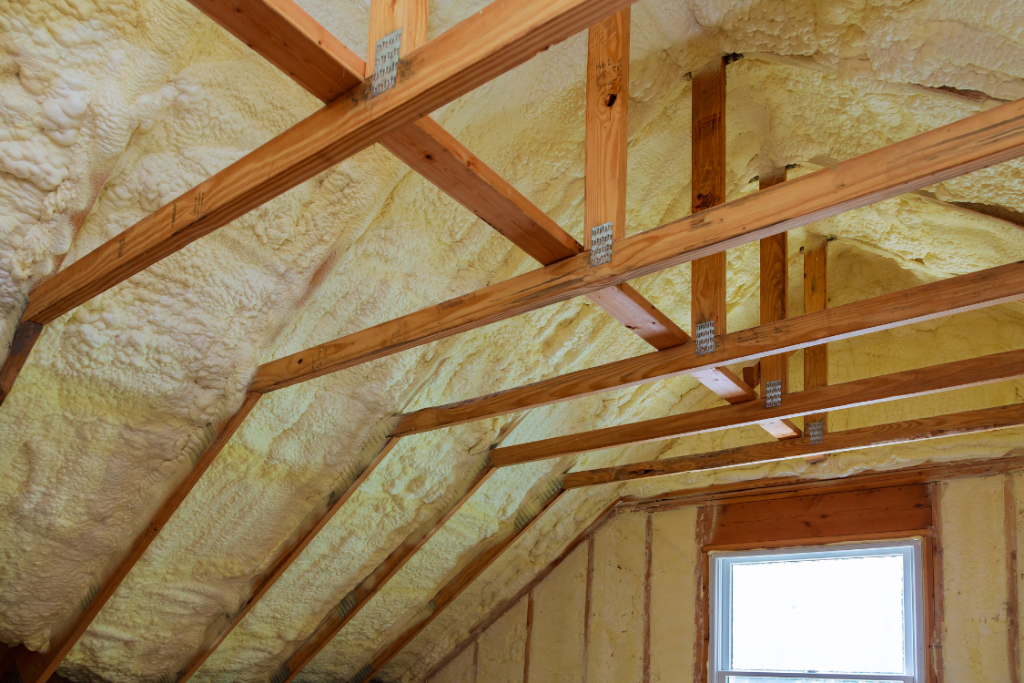
Effective air sealing, vapour control, and shading systems reduce HVAC demand and improve passive performance. Accurate thermal modelling (e.g., using NatHERS or JV3 of the NCC) is essential to assess and optimise envelope performance.
Compliance Pathways and Benchmarking
In Australia, HVAC energy efficiency is enforced through:
- NCC Section J Part J5 for HVAC system performance, requiring minimum fan, pump, chiller, and boiler efficiencies.
- Green Star Energy Credit 15, rewarding high-performing HVAC systems integrated with renewable energy.
- NABERS Energy certification, where HVAC operation directly affects base building and tenancy ratings.
Projects may follow Deemed-to-Satisfy (DtS) paths for compliance or pursue JV3 performance solutions using whole-building energy simulation. Accurate energy modelling and documentation are essential for demonstrating equivalency, particularly for complex HVAC designs or unconventional building envelopes.